 |
Physics Update
All-optical trapping of a degenerate Fermi gas has been demonstrated. First created in a magnetic trap (see Physics Today, October 1999, page 17),
a degenerate Fermi gas consists of fermionic atoms--those with an odd total
number of protons, neutrons, and electrons--sufficiently dense and cold that
only the lowest trap energy levels are occupied. An all-optical trap has
previously been used to confine a Bose-Einstein condensate (see Physics Today, July 2001, page 20 and September 2001, page 79). Now, using a stable, high-power CO2
laser, physicists at Duke University have created a kind of "optical bowl"
for lithium-6 atoms: Slowly lowering the bowl's rim permitted the hottest
atoms to evaporate. The researchers then adiabatically recompressed the trap
to its full depth, which tightly confined the remaining degenerate gas. In
this way, an equal mixture of lithium atoms in spin-up and spin-down states
was captured, a feat not possible in magnetic traps. According to the Duke
researchers, such equal two-state mixtures are potentially ideal for forming
neutrally charged, quasibound pairs of atoms in Fermi gases--something the
researchers hope to observe soon. Several groups are pursuing such an atomic-gas
analog of superconductivity in different ways. (S. R. Granade et al., Phys. Rev. Lett. 88, 120405, 2002.) --bps
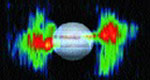 |
Jupiter's magnetosphere has been simultaneously sampled by two spacecraft,
Galileo (already on patrol in the Jupiter system) and Cassini-Huygens (headed
toward Saturn). Just as Cassini was approaching Jupiter in January 2001,
other Earthbound observatories, including radio telescopes and the Hubble
(optical) and Chandra (x-ray) satellites, were turned to the giant planet.
The Sun also cooperated: Three interplanetary shock waves in the solar wind
swept by. The two spacecraft caught Jupiter's magnetosphere in the act of
being compressed. That compression produced strong electric fields and therefore
particle accelerations, which brightened Jupiter's auroras. Internal magnetospheric
dynamics caused other observed auroral brightenings and a wind of neutral
atoms--formed from ions spewed by Io's volcanic eruptions--sent outward against
the incoming solar wind. Such energetic neutral atoms had not been directly
observed before. The flyby also provided the first opportunity to observe
electrons above 50 MeV trapped in Jupiter's radiation belts. Shown here is
the synchrotron radiation from those electrons, with a superimposed Hubble
image of Jupiter. (Seven articles in Nature 415, 985 ff, 2002.) --pfs
Microtesla nuclear magnetic resonance (NMR) has been demonstrated.
In conventional NMR, a several-tesla magnetic field is used to orient atomic
nuclei in the sample. The polarized nuclei can resonantly absorb a burst
of radio waves, and precess around the imposed field. The spectral "chemical
shift" information from reemitted radio waves is then used to identify molecules.
NMR also lies at the heart of magnetic resonance imaging (MRI). Now, a team
of scientists led by John Clarke and Alexander Pines (Lawrence Berkeley National
Laboratory and the University of California, Berkeley) have exploited an
often overlooked fact: For a homogeneous field, the NMR linewidth scales
linearly with the field strength. Thus, a 1000-fold reduction in field strength
produces a line both narrower and taller by that same factor. The researchers
placed a small liquid sample of methanol and phosphoric acid in a polarizing
field of only 1 mT and a much weaker orthogonal measuring field of 5 mT (Earth's field is roughly 50 mT).
The group then turned off the polarizing field and used a SQUID to detect
not chemical shift but "J-coupling," which can measure an atom's chemical
environment as well as its identity. In that way, they not only identified
protons and phosphorous-31, but saw the signature--a doublet split by 10.4
Hz--of the covalent bonds in trimethyl phosphate. These techniques open the
possibility for "pure J" spectroscopy and perhaps could form the basis of
inexpensive MRI machines. (R. McDermott et al., Science 295, 2247, 2002.) --pfs
Electrical biosensors for individual living cells were described at
the March meeting of the American Physical Society. Cells are complex networks
of interacting molecules, and are usually studied with optical techniques.
Electrical measurements, however, can provide complementary information.
Toward that end, Lydia Sohn of Princeton University described several new
biosensors. With one, she measured the amount of DNA in a single living cell
passed through a small fluid chamber between two metal electrodes. The cell
changed the system's capacitance in a way that reflected the amount of the
cell's negatively charged DNA but not its other ions. Sohn reported that
the technique can identify the stage of a cell's development (since cells
can contain different amounts of DNA at different stages) and can potentially
distinguish cancerous cells from healthy ones. Sohn also described a biosensor
that can detect small amounts of a specific protein in live E. coli
cells. The eventual goal of Sohn's lab is to take inventory of a living cell's
protein contents--something that cannot be done with current protein assay
techniques, which require the destruction of cells. (http://www.aps.org/meet/MAR02/baps/vpr/layf7-003.html.) --bps
© 2002 American Institute of Physics
|
 |
|